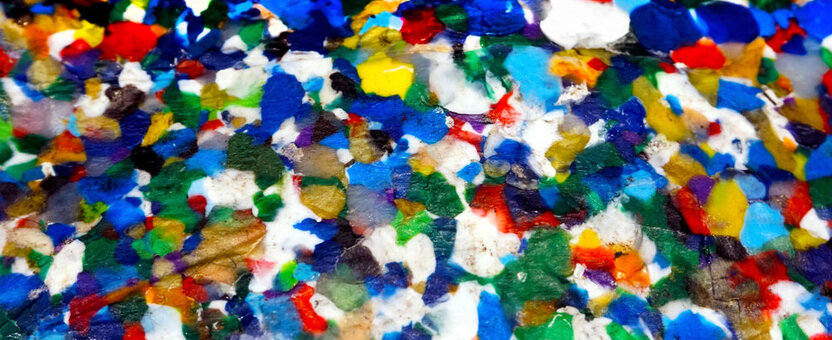
2023
Guest commentary, What’s new
“Advanced Recycling” of Plastics: Largely waste disposal by another name (Part 2)
“Advanced recycling,” also sometimes called molecular recycling or chemical recycling, is a term that encompasses several technologies that use heat, solvents, enzymes, or microwaves to break down plastic waste. Among the so-called “advanced recycling” methods, attempts to commercialize and scale up have focused mainly on pyrolysis and gasification. Pyrolysis or gasification of plastics both pose significant challenges, and their performance thus far has been poor.
Mixed feedstocks, contaminants, & other challenges
As noted in Part 1, pyrolysis decomposes plastics or other materials at moderately elevated temperatures in an oxygen-free or low-oxygen environment, leading the plastics to de-polymerize. The products of pyrolysis are syngas, pyrolysis oil, and char, as well as carbon monoxide and hydrogen. Gasification converts plastics into gases and is often preceded by pyrolysis.
Most pyrolysis operations intended to recycle plastics at scale into fuels or resins for new plastics have failed to meet expectations, and many have closed because they were not commercially viable. This is largely due to the real-world composition of plastic waste, which includes multiple polymer types and an array of additives and contaminants.
According to one recent industry article, variability of feedstock poses a particular challenge: “The different polymers that are fed into a pyrolysis reactor break along different patterns. In particular, molecules with high degrees of branching crack more easily than linear ones.”
Polyvinyl chloride (PVC) and polyethylene terephthalate (PET) are particularly problematic and need to be removed from the waste stream prior to pyrolysis. PVC yields hydrochloric acid (HCl) when pyrolyzed. HCl is corrosive to equipment, and chlorinated compounds contaminate and degrade the final liquid product.
PET contains oxygen and much is converted into carbon dioxide. Condensates also reportedly contaminate the equipment. Ongoing research with various catalysts is attempting to address problems associated with pyrolysis of PET. Eastman Chemicals is investing heavily in methanolysis, which uses methanol at high temperatures and pressures to de-polymerize polyethylene terephthalate (PET) with the intent of making new products. Pigments, dyes, paper, metal fragments, and other contaminants also affect the quality of the final pyrolysis products.
Similarly, products of gasification are affected by both the composition of the plastic waste stream and the design of the gasification system. As with pyrolysis, PVC plastic needs to be removed from the waste stream prior to processing since significant amounts result in production of corrosive hydrochloric acid.
Are pyrolysis and gasification really just incineration?
By definition, incineration requires combustion or burning — a high temperature chemical reaction between a fuel and an oxidant (usually atmospheric oxygen) that gives off heat. Waste incinerators, which combust waste at high temperatures (sometimes in waste-to-energy plants) are regulated by the US EPA under the Clean Air Act.
Promoters of pyrolysis and gasification often argue that these processes are different from incineration and the facilities should not be regulated as incinerators. Their rationale usually emphasizes the stage of the processes conducted in an oxygen-free or low-oxygen environment where the waste material does not burn but rather melts into component parts.
But most existing facilities are largely producing fuels that are then burned, rather than producing new plastics. Syngas is often burned in the same facility to heat the pyrolysis chamber. Pyrolysis oils are typically only suitable to burn as low-value fuels rather than being used as plastic feedstocks.
Moreover, the residual char and melted plastic that are not converted to syngas or pyrolysis oil are typically incinerated as well. Thus, incineration is involved at various stages within these technologies.
Limitations of life cycle assessments
Numerous life cycle assessments (LCAs) have investigated the environmental impacts of end-of-life treatments of plastic waste, including pyrolysis and gasification. LCAs are heavily dependent on assumptions about a variety of factors, including the composition of the waste stream and boundary conditions that determine the extent of the analysis. Variation in these assumptions lead to stark differences in the results of the assessments.
For example, higher quality oils (e.g. naphtha) from pyrolysis could theoretically be used as feedstock for plastics or solvents. This has rarely been accomplished at scale. However, an LCA that assumes a substantial output of high-quality naphtha will look much more favorable than one based on an output of low-quality fuel that cannot be used to make new products and can only be burned for energy.
Criteria for evaluating plastic waste management technologies
The main challenges for commercial scaling of pyrolysis or gasification of plastic waste are the inconsistent quality of feedstock, inefficient and costly sorting, non-existent markets for poorly-standardized products, and unclear regulations around plastic waste management.
Institutions, such as health care facilities, that wish to manage plastic waste better should start by evaluating opportunities to reduce their use of plastic. This includes considering what is truly essential, reusing products when possible, and mechanically recycling what is unavoidable.
In choosing a technology for managing unavoidable plastic waste, institutions can use some of the same criteria they would use for evaluating other processes and products. These include:
- Health and safety for workers and communities. For example, does the technology require waste sorting, separation, and removal of certain polymers such as PVC or PET? Who will do it? How does performance of the technology vary with changing composition of the waste stream? How durable is the technology over time? What’s the evidence?
- Climate and ecosystem impacts. For example, what are the energy requirements for operation? What is the source of energy? Does energy consumption change with composition of the waste stream? What are the emissions to air and water from facilities managing the waste?
- Environmental justice concerns. What is the demographic make-up of the community where the facility is located?
- Circularity. To what extent are the products of chemical recycling truly circular in the economy? Are they simply down-cycled, used once in a new product, and then disposed of? Are they quickly lost to combustion?
- Transparency of processes, products. Are vendors of specific pyrolysis or gasification technologies able and willing to provide full and complete answers to these questions?
“Advanced recycling” technologies have overwhelmingly failed to perform as promised. They are a diversion to keep us from confronting the profound public and environmental health impacts of global plastic production, projected to increase steadily from about 391 million metric tons (MMT) in 2021 to almost 600 MMT in 2050.
As fossil fuels for energy slowly give way to cleaner energy sources, oil and gas industries are betting that this growth in plastic production will be a winning wager for maintaining their enormous profits. Plastic waste already pollutes virtually all land, air, sea, and freshwater ecosystems throughout the world. It’s long past time to begin turning off the tap.
This is the second in a two-part series on so-called “advanced recycling” of plastics. Also see: “Advanced Recycling” of Plastics: Largely waste disposal by another name (Part 1).
This series is excerpted and condensed from a white paper written for Health Care Without Harm.
Ted Schettler, MD, MPH is Science Director for the Science and Environmental Health Network and a member of the CHE Advisory Team. He has worked extensively with community groups and non-governmental organizations throughout the US and internationally, addressing many aspects of human health and the environment, and has served on advisory committees of the US EPA and National Academy of Sciences. His publications include Generations at Risk: Reproductive Health and the Environment; In Harm's Way: Toxic Threats to Child Development; and Environmental Threats to Healthy Aging, as well as numerous articles in the medical literature.